A new study published in the journal Buildings reviews Denmark’s current passive heating strategies and examines the suitability of such measures for the design of a future building.
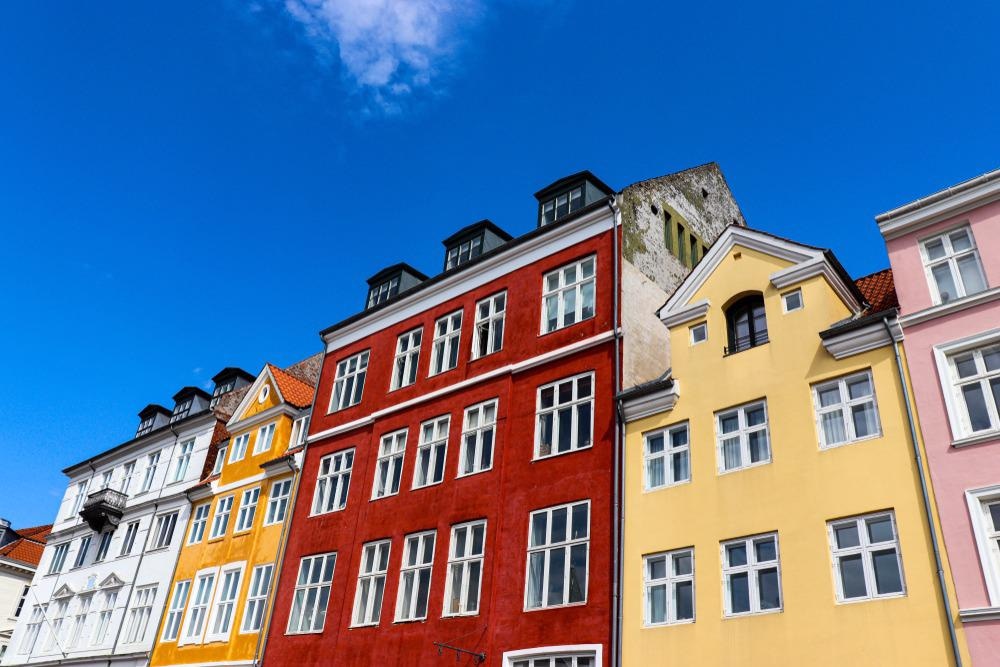
Study: Bioclimatic Architecture Strategies in Denmark: A Review of Current and Future Directions. Image Credit: natalia gh/Shutterstock.com
The average temperatures of European and Nordic countries have been rising since the pre-industrial period, and they are expected to increase even more. The rise in annual average temperature reduces a building’s demand for heating, especially during winters.
However, increasing average temperatures, more recurrent heatwaves, and nights with more than 20 °C will also increase the requirement for cooling to fulfill the thermal comfort of occupants.
The consequent enhanced cooling energy is at odds with the current effort of the EU to gain climate neutrality. Hence, the requirement for actions to counteract such climate effects is obvious.
This paper reviews Denmark’s current passive heating strategies and examines the suitability of such measures for the design of a future building. Further, the result of the study is complemented by analyzing 25 bioclimatic architecture-based building design projects in Denmark to offer an understanding of the real application of the strategies identified in the ongoing practice of building design.
In such a process, the bioclimatic architecture is mainly characterized by detecting the passive heating and cooling strategies. Then, for each passive strategy, search keywords are mentioned and employed to perform the systematic literature study. After which, the bioclimatic strategies implemented in the 25 actual building projects are defined and examined, and finally, the findings are presented and discussed.
Bioclimatic architecture is the design of a building that is made suitable to the local climate with passive strategies in order to gain a favorable indoor environmental quality with the least energy consumption possible.
Strategies might involve passive heating, which is heat retention and heat admission, and passive cooling, which is heat exclusion and heat dissipation, in a bioclimatic context. An outline of the passive cooling and heating strategies is illustrated in Figure 1. The passive strategies are further overviewed, and keywords for consequent literature searches are obtained.
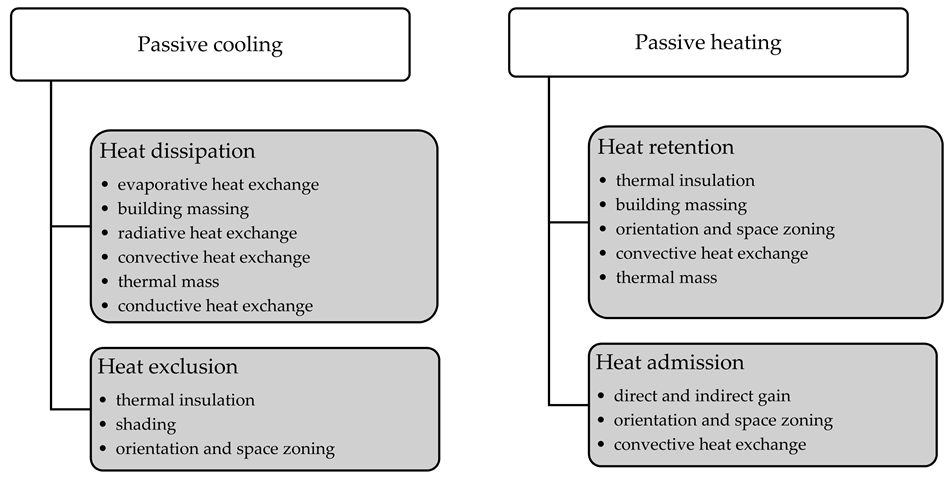
Figure 1. Bioclimatic architecture strategies—passive cooling and passive heating. Based on information obtained from Košir, extended using Lechner and La Roche. Image Credit: Bugenings and Kamari, 2022
After the analysis of the site analysis and local climate conditions, the first step in passive solar design is to identify the building’s orientation. Attention must be given to on-site shading or other aspects, like transparent areas, building shape, or insulation levels that will impact design stages later (see Figure 2).
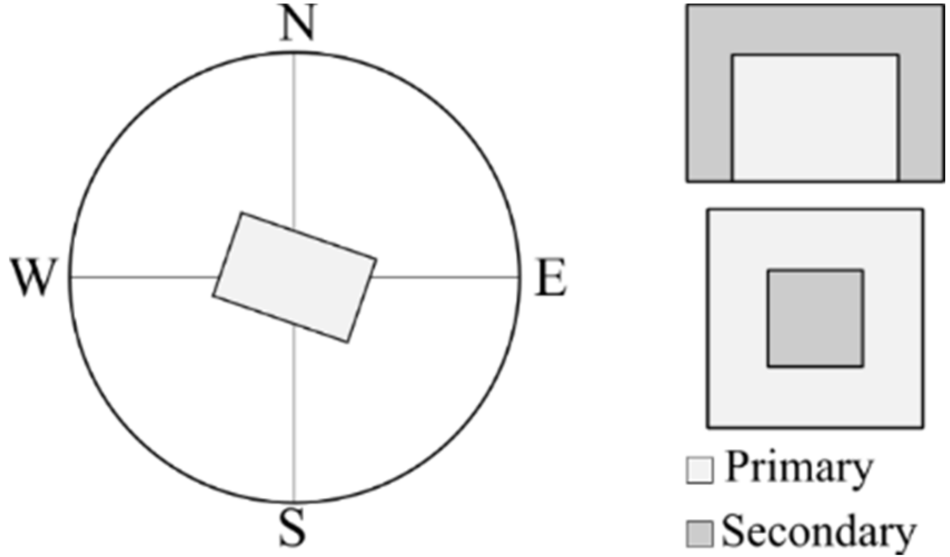
Figure 2. Building orientation (left) and space zoning (right). Image Credit: Bugenings and Kamari, 2022
Defining the shape of a building is a key part of the initial design process. This comprises the compactness and aspect ratio of the buildings. The more compact a building gets, the lesser the heat losses and vice versa (see Figure 3).
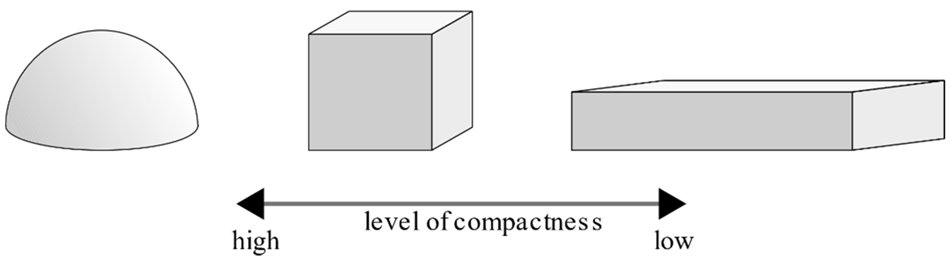
Figure 3. Building massing—level of compactness. Image Credit: Bugenings and Kamari, 2022
The thermal mass of the building can store or expel heat. Hence, it can be used for both passive heating and cooling. In addition to conventional building materials, phase-change materials (PCM) could be employed to enhance the thermal mass of a building (see Figure 4).
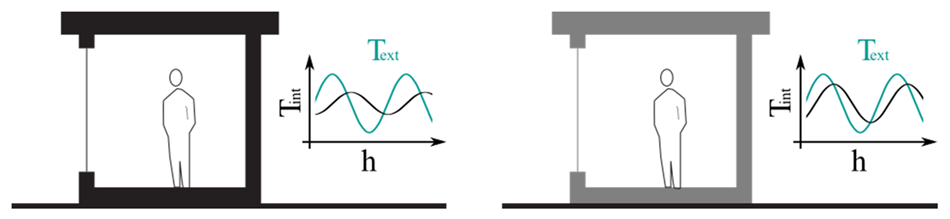
Figure 4. Thermal mass—heavy (left) and light construction (right). Image Credit: Bugenings and Kamari, 2022
One of the important strategies to avoid heat losses is thermal insulation. In temperate and cold climates, previous and current legislation has been concerned to decrease the demand for heating by insulating and air tightening the thermal envelope. It, thus, achieves a low heat transfer coefficient (U)—a measure for the heat loss per area of particular building components (Figure 5).
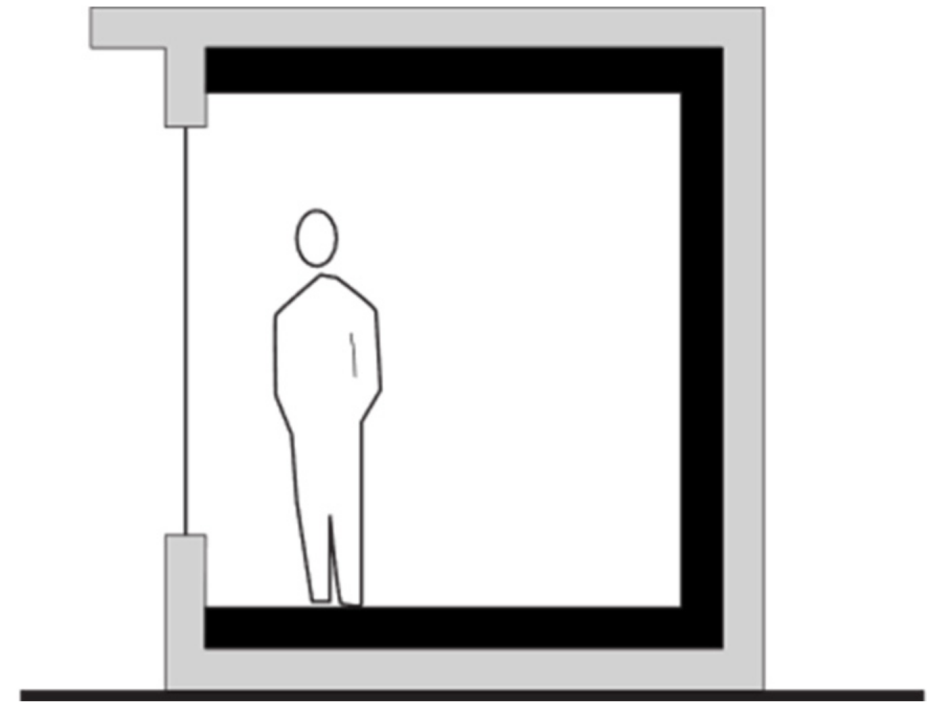
Figure 5. Thermal insulation. Image Credit: Bugenings and Kamari, 2022
Employing solar radiation is the easiest method to heat the building. It can be done indirectly when a Trombe wall or a sunspace is heated up, and warm air is made to circulate through the building (see Figure 6).
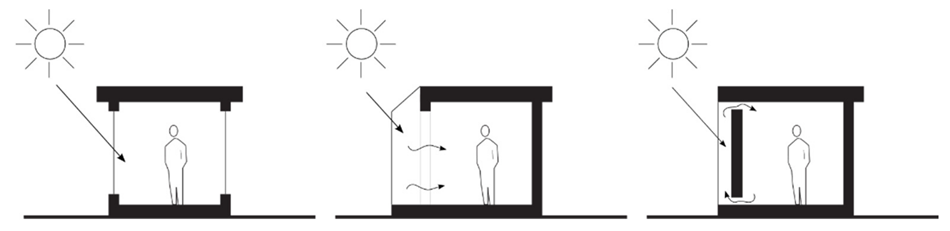
Figure 6. Direct and indirect gains—glazing (left), sunspace (middle), and solar/Trombe wall (right). Image Credit: Bugenings and Kamari, 2022
Dedicated shading tools are categorized into fixed and moveable shading defined by the color, the material used, and the position that is relative to the opening (see Figure 7).
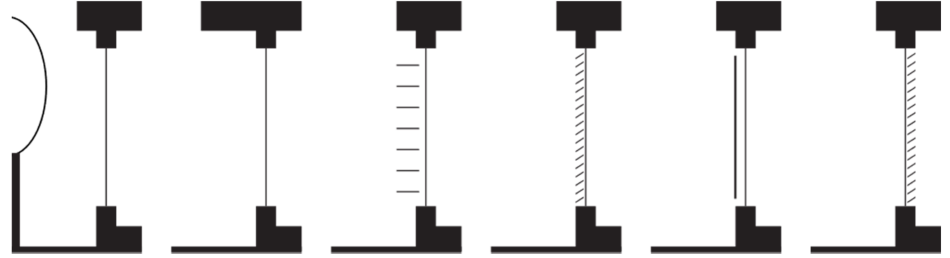
Figure 7. Solar shading—F.L.T.R. Vegetation, overhang, fixed horizontal slats, external venetian blind, external screen, and internal venetian blind. Image Credit: Bugenings and Kamari, 2022
When the indoors is too warm, eventually compromising comfort, the air circulating near the occupants might be raised. This can be performed with natural ventilation techniques, usually divided into cross and stack ventilation, single-sided, and windcatchers/wind towers (see Figure 8).

Figure 8. Natural ventilation—single-sided ventilation (left), cross ventilation (middle) and stack ventilation (right). Image Credit: Bugenings and Kamari, 2022
With relatively stable temperatures, the soil might provide a cooling and heating likelihood for a building. Eventually, earth sheltering could cut off outside air infiltration, offer extra thermal resistance, and decrease solar and convective heat gains (see Figure 9).
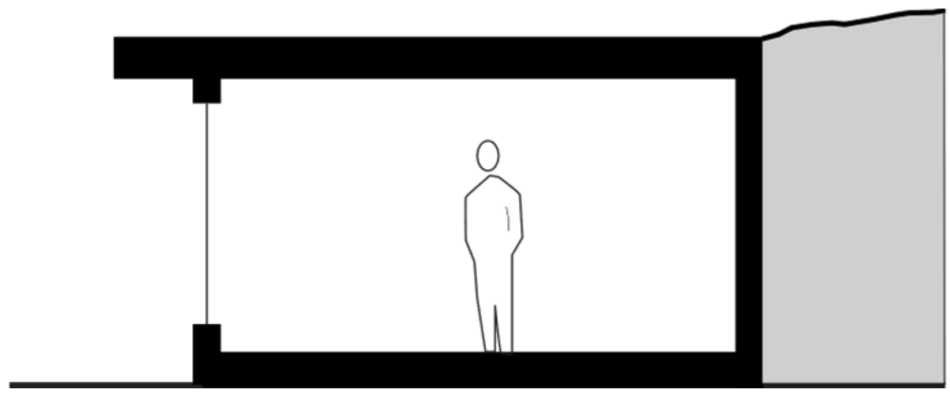
Figure 9. Conductive heat exchange. Image Credit: Bugenings and Kamari, 2022
Here, the concrete serves as a sink, cooling the building. Rather than concrete, water bags could also be used (see Figure 10).
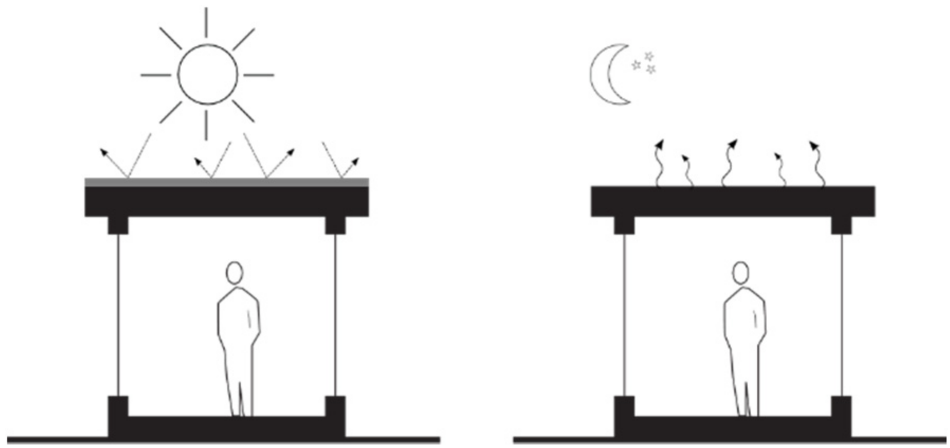
Figure 10. Radiative cooling—day (left) and night (right). Image Credit: Bugenings and Kamari, 2022
The technique functions suitably well in hot and dry climates, whereas its efficiency is reduced in humid and cold climates owing to the already increased water saturation of the air (see Figure 11).
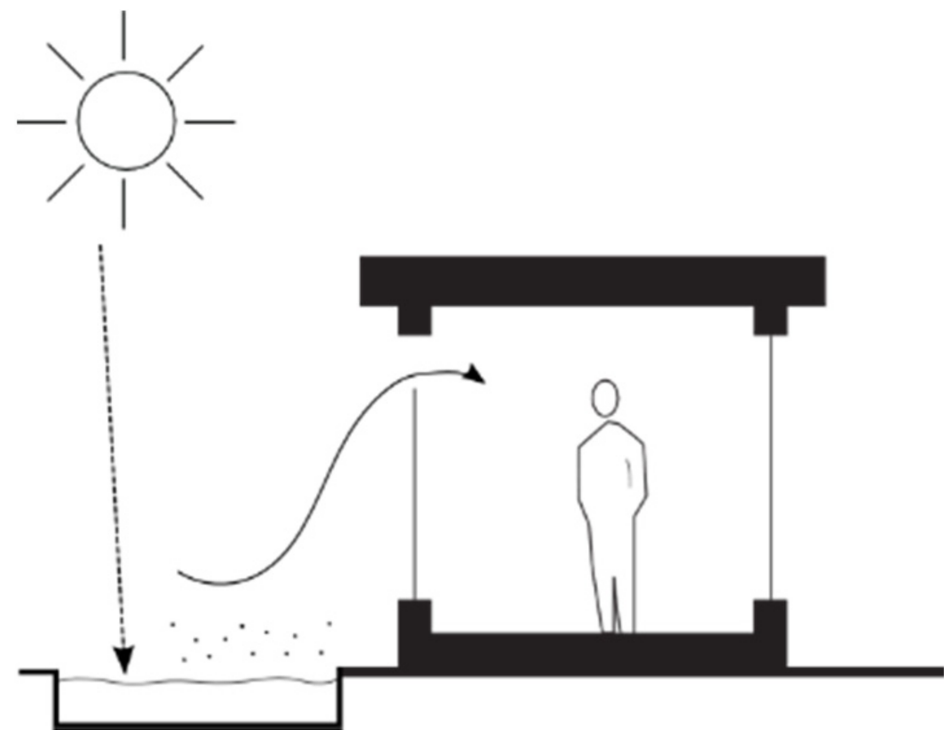
Figure 11. Evaporative cooling. Image Credit: Bugenings and Kamari, 2022
Methodology
Firstly, a literature review is performed to offer an outline of already investigated passive strategies in Denmark. Secondly, a collection of Danish building projects using passive strategies is presented. The literature review findings aim to present an all-inclusive outline of all existing investigations, whereas the presentation of the Danish building projects shows data only from a selection of past years.
Additionally, a citation search of the associated publications was performed. The search is documented with the upgraded PRISMA (Preferred Reporting Items for Systematic Reviews and Meta-Analyses) flowchart. For every search of a passive strategy, one PRISMA diagram was formed. Figure 12 illustrates one such example.
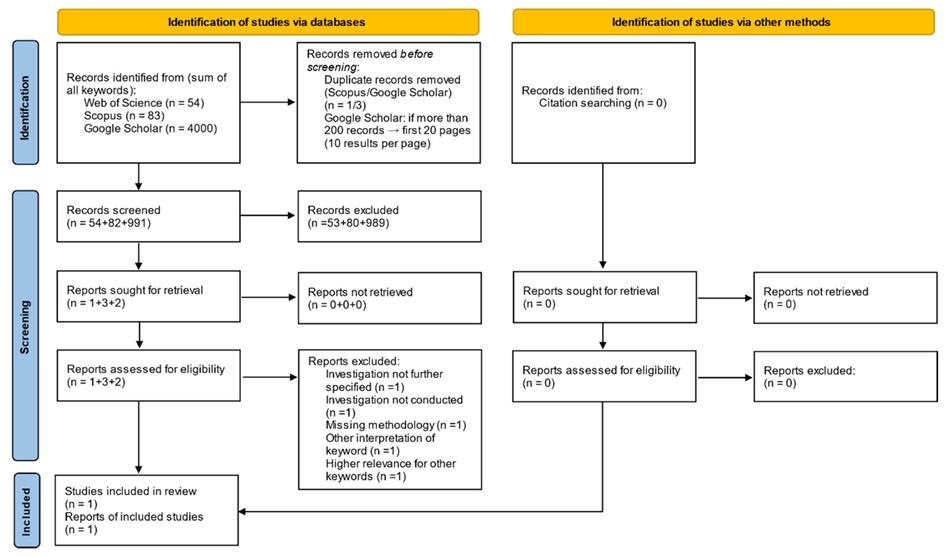
Figure 12. Example PRISMA diagram for keywords “orientation”, “building layout,” and “space zoning”. Image Credit: Bugenings and Kamari, 2022
Only the bioclimatic architectural factors that are specified within the explanation of the project are established. All projects are defined by the keywords.
Results
A total of 49 (from 52 different investigations) publications were identified to be relevant for the examination of Denmark’s bioclimatic architectural strategies.
The reviewed strategies included thermal insulation, thermal mass, and natural ventilation as the most studied passive strategies, and conductive heat and space zoning exchange as the least (Figure 13).
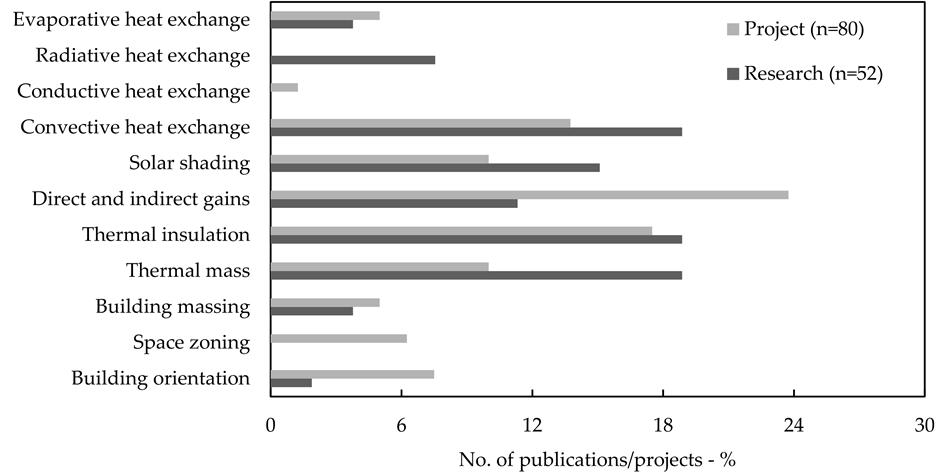
Figure 13. Comparison of the number of investigated and built passive strategies. Image Credit: Bugenings and Kamari, 2022
Additionally, the review showed that the research interest in passive cooling began in 2007, just after the new Danish building regulations entered into force in 2006 (see Figure 14).
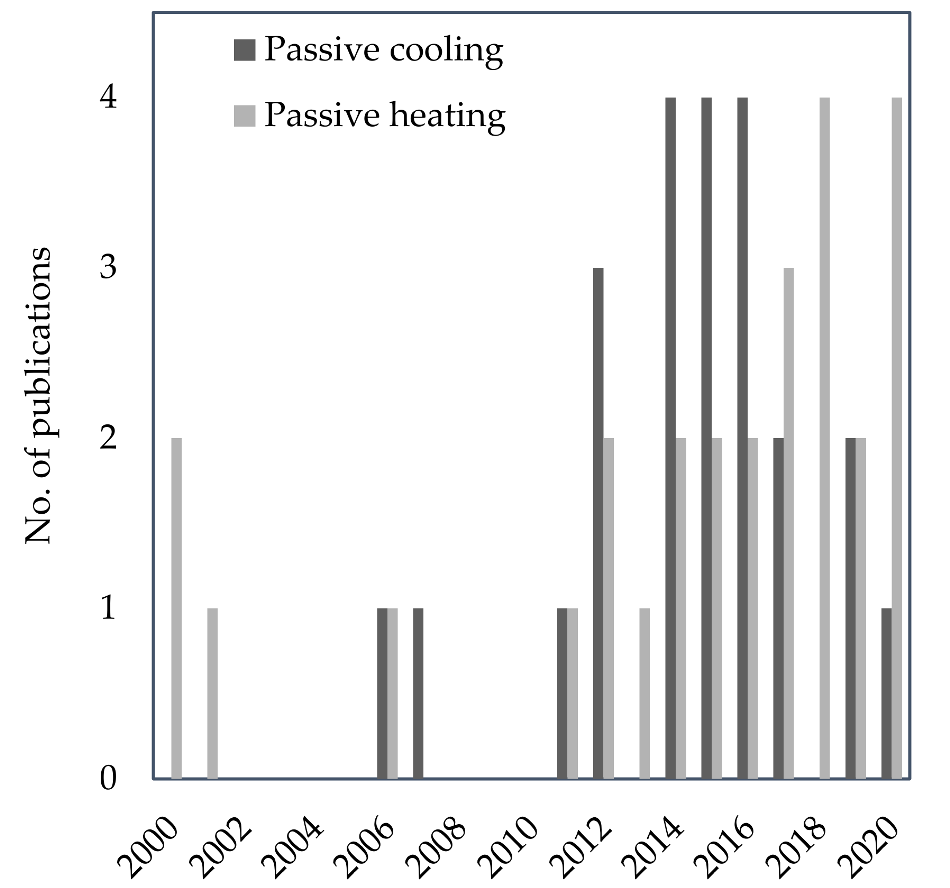
Figure 14. Number of publications per year. Image Credit: Bugenings and Kamari, 2022
A total of 25 building projects were reviewed. Space zoning was never examined but specified multiple times as a scheme for built projects. Building massing was examined in the urban context, where the considerable impact on energy consumption was shown.
For new buildings and renovations, windows were studied. Atria were applied for residential as well as non-residential buildings. On contrary, sunspaces were only implemented in residential buildings.
The solar shading effect was examined in terms of energy consumption and thermal comfort for office and residential buildings. Natural ventilation was among the most researched ones. Radiative cooling was researched along with unglazed solar collectors and photovoltaic/thermal panels.
Conclusions
This study showed an elaborate systematic review of theoretical studies examining bioclimatic architecture that is commonly used in the Danish climate, with an outline of 25 actual building projects present in Denmark. The result is that, in Denmark, almost all recognized passive strategies are employed, while the concentration is on passive heating strategies.
The studies about direct and indirect gains, which were investigated and applied mainly in residential buildings, will most probably give attention to attaining a balance between contributing to the reduction in heating demand and an increase in cooling demand.
They may be largely examined and implemented along with solar shading. In addition, their significance to residential buildings will remain the same as today’s, while the significance for non-residential buildings will remain low, as it is at present.
Although the literature search was performed to the best of the author’s knowledge, it would still have drawbacks that need to be recognized when this article into account. The search was performed in English, looking at publications written in English alone. As Denmark is the focus of this study, there is a possibility for identical investigations to be performed in Danish.
Journal Reference:
Bugenings, L. A. & Kamari, A. (2022) Bioclimatic Architecture Strategies in Denmark: A Review of Current and Future Directions. Buildings, 12(2), 224. Available online: https://www.mdpi.com/2075-5309/12/2/224/htm
References and Further Reading
- The European Environment Agency (2021) Global and European Temperatures. Available at: https://www.eea.europa.eu/data-and-maps/indicators/global-and-european-temperature-10/assessment.
- Ministry of Environment of Denmark (2021) The Weather in Denmark will Get Warmer, Wetter and Wilder. Available at: https://en.klimatilpasning.dk/knowledge/climate/denmarksfutureclimate/.
- World Meteorological Organization (2021)State of the Global Climate 2020; Report No. 1264; World Meteorological Organization: Geneva, Switzerland. Available at: http://resp.llas.ac.cn/C666/handle/2XK7JSWQ/323706.
- Marsh, R., et al. (2010) Housing and energy in Denmark: Past, present, and future challenges. Building Research & Information, 38(1), pp. 92–106. doi.org/10.1080/09613210903226608.
- Marsh, R., et al. (2010) Towards a New Paradigm: Design Strategies for Architecture, Energy and Climate Change using Danish Office Buildings as a Case Study. Nordic Journal of Architectural Research, 22.
- Ministry of Environment of Denmark (2021) Climate Change Impact on Buildings and Constructions. Available at: https://en.klimatilpasning.dk/sectors/buildings/climate-change-impact-on-buildings/.
- Aghimien, E. I., et al. (2021) Bioclimatic architecture and its energy-saving potentials: A review and future directions. Engineering, Construction and Architectural Management. doi.org/10.1108/ECAM-11-2020-0928.
- Cabeza, L F & Chàfer, M (2020) Technological options and strategies towards zero energy buildings contributing to climate change mitigation: A systematic review. Energy and Building, 219, p. 110009. doi.org/10.1016/j.enbuild.2020.110009.
- Manzano-Agugliaro, F., et al. (2015) Review of bioclimatic architecture strategies for achieving thermal comfort. Renewable and Sustainable Energy Reviews, 49, pp. 736–755. doi.org/10.1016/j.rser.2015.04.095.
- Marsh, R (2012) The Paradox of Climate Change Mitigation and Adaptation in Danish Housing. Open House International, 37(4), pp. 19–28. doi.org/10.1108/OHI-04-2012-B0003.
- Watson, D (2013) Bioclimatic Design. In: Sustainable Built Environments; Loftness, V., Haase, D., Eds.; Springer: New York, NY, USA. Available at: https://link.springer.com/content/pdf/10.1007/978-1-0716-0684-1_225.pdf.
- Košir, M (2019) Climate Adaptability of Buildings—Bioclimatic Design in the Light of Climate Change; Springer International Publishing: Cham, Switzerland. Available at: https://link.springer.com/book/10.1007/978-3-030-18456-8?noAccess=true.
- Lechner, N (2015) Heating, Cooling, Lighting—Sustainable Design Methods for Architects, 4th ed.; John Wiley & Sons, Inc.: Hoboken, NJ, USA.
- La Roche, P (2017) Carbon-Neutral Architectural Design, 2nd ed.; CRC Press—Taylor & Francis Group: Boca Raton, FL, USA. doi.org/10.1201/9781315119649.
- Olgyay, V., et al. (2015) Design with Climate—Bioclimatic Approach to Architectural Regionalism; Princton Universtiy Press: Princeton, NJ, USA.
- Pacheco-Torres, R., et al. (2012) Energy efficient design of building: A review. Renewable and Sustainable Energy Reviews, 16(6), pp. 3559–3573. doi.org/10.1016/j.rser.2012.03.045.
- Gupta, N &Tiwari, G N (2016) Review of passive heating/cooling systems of buildings. Energy Science &Engineering, 4(5), pp. 305–333. doi.org/10.1002/ese3.129.
- Aksoy, U T & Inalli, M (2006) Impacts of some building passive design parameters on heating demand for a cold region. Building and Environment, 41(12), pp. 1742–1754. doi.org/10.1016/j.buildenv.2005.07.011.
- Song, Y.L., et al. (2021) A review on conventional passive cooling methods applicable to arid and warm climates considering economic cost and efficiency analysis in resource-based cities. Energy Reports, 7, pp. 2784–2820. doi.org/10.1016/j.egyr.2021.04.056.
- Amirifard, F., et al. (2018) Application of passive measures for energy conservation in buildings—A review. Advances in Building Energy Research, 13(2), pp. 282–315. doi.org/10.1080/17512549.2018.1488617.
- Johnsen, K & Winther, FV (2015) Dynamic Facades, the Smart Way of Meeting the Energy Requirements. Energy Procedia, 78, pp. 1568–1573. doi.org/10.1016/j.egypro.2015.11.210.
- Chan, H.Y., et al. (2010) Review of passive solar heating and cooling technologies. Renewable and Sustainable Energy Reviews, 14(2), pp. 781–789. doi.org/10.1016/j.rser.2009.10.030.
- Geetha, N B &Velraj, R (2012) Passive cooling methods for energy efficient buildings with and without thermal energy storage–A review. Energy Education Science and Technology Part A: Energy Science and Research, 29, 913–946. Available at: https://www.semanticscholar.org/paper/Passive-cooling-methods-for-energy-efficient-with-A-Geetha-Velraj/eabe66ea2769d2651e5c688ac24d1aa6ca685a42
- Chetan, V., et al. (2020) Review of Passive Cooling Methods for Buildings. Journal of Physics: Conference Series, 1473, p. 012054. doi.org/10.1088/1742-6596/1473/1/012054.
- Ahmed, T., et al. (2021) Natural ventilation in warm climates: The challenges of thermal comfort, heatwave resilience and indoor air quality. Renewable and Sustainable Energy Reviews, 138, p. 110669. doi.org/10.1016/j.rser.2020.110669.
- González, A.T., et al. (2016) Assessing the applicability of passive cooling and heating techniques through climate factors: An overview. Renewable and Sustainable Energy Reviews, 65, pp. 727–742. doi.org/10.1016/j.rser.2016.06.077.
- Kamal, MA (2012) An Overview of Passive Cooling Techniques in Buildings: Design Concepts and Architectural Interventions. Acta TechnicaNapocensis: Civil Engineering & Architecture, 55(1), pp. 84–97.
- Cuce, P M & Riffat, S (2016) A state of the art review of evaporative cooling systems for building applications. Renewable and Sustainable Energy Reviews, 54, pp. 1240–1249. doi.org/10.1016/j.rser.2015.10.066.
- Page, M. J., et al. (2021) PRISMA 2020 explanation and elaboration: Updated guidance and exemplars for reporting systematic reviews. BMJ, 372, p. n160. doi.org/10.1136/bmj.n160.
- Liberati, A., et al. (2009) The PRISMA statement for reporting systematic reviews and meta-analyses of studies that evaluate healthcare interventions: Explanation and elaboration. BMJ, 339, p. b2700. doi.org/10.1136/bmj.b2700.
- Zukowska-Tejsen, D., et al. (2019) Solar control solutions for reducing overheating risksin retrofitted Danish apartment buildings from the period 1850–1900—A simulation-based study. E3S Web of Conferences, 111, p. 03051. doi.org/10.1051/e3sconf/201911103051.
- Kazanci, O B & Olesen, B W (2016) Beyond nZEB: Experimental investigation of the thermal indoor environment and energy performance of a single-family house designed for plus-energy targets. Science and Technology for the Built Environment, 22, pp. 1024–1038. doi.org/10.1080/23744731.2016.1208001.
- Dabaieh, M & Eybye, B T (2016) A comparative study of human aspects in acclimatization of adobe vernacular architecture: A case from Denmark and Egypt. A/Z Journal - İTÜ Faculty of Architecture, 13, pp. 29–41.
- Hummelshøj, R M & Rahbek, J E (2000) “ECO-HOUSE 99”-Full-Scale Demonstration of Solar Walls with Building Integrated Heat Storages. In Proceedings of the 3rd ISES European Solar Congress, Copenhagen, Denmark, 19–22 June.
- Mikkelsen, S (2018) umanistic Architecture through Innovation. In Sustainability in Scandinavia—Architecture Design and Planning; Malkawi, A., Beim, A., Stenberg, E., Nygaard, M., Eds.; Edition Axel Menges: Fellbach, Germany.
- Beim, A., et al. (2002) Økologi og Arkitektonisk Kvalitet; Kunstakademiets Arkitektskole, Institut 1: Copenhagen, Denmark.
- Ehmann, S., et al. (2014) Building Better: Sustainable Architecture for Family Homes; Gestalten Verlag: Berlin, Germany.
- Dirckinck-Holmfeld, K (1994) Økologisk Byggeri i Danmark—Danish Ecological Building; Arkitektens Forlag: Copenhagen, Denmark.
- Henning Larsen Architects (2012) Design with Knowledge; Kongebro, S., Henning Larsen Architects, Eds.; Henning Larsen Architects: Copenhagen, Denmark, 2012; Available at: https://issuu.com/.
- Sattrup, P A (2013) Strømann-Andersen, J. Building typologies in Northern European cities: Daylight, solar access, and building energy use. Journal of Architectural and Planning Research, 30, pp. 56–76.
- Strømann-Andersen, J & Sattrup, P A (2011) The urban canyon and building energy use: Urban density versus daylight and passive solar gains. Energy and Buildings, 43, pp. 2011–2020. doi.org/10.1016/j.enbuild.2011.04.007.
- Møller, C F (2021) Danmark A/S. LEGO® Campus. Available at: https://www.cfmoller.com/p/LEGO-Campus-i3355.html
- Foteinaki, K., et al. (2016) Modeling Energy Flexibility of Low Energy Buildings Utilizing Thermal Mass. In Proceedings of the 9th International Conference on Indoor Air Quality, Ventilation & Energy Conservation in Buildings, Seoul, Korea, pp. 23–26 October.
- Le Dréau, J & Heiselberg, P (2016) Energy flexibility of residential buildings using short term heat storage in the thermal mass. Energy, 111, pp. 991–1002. doi.org/10.1016/j.energy.2016.05.076.
- Marsh, R., et al. (2001) Passive solar energy and thermal mass: The implications of environmental analysis. arq: Architectural Research Quarterly, 5, pp. 79–89. doi.org/10.1017/S1359135501001075.
- Hagenau, M & Jradi, M (2020) Dynamic modeling and performance evaluation of building envelope enhanced with phase change material under Danish conditions. Journal of Energy Storage, 30, p. 101536. doi.org/10.1016/j.est.2020.101536.
- Hu, Y., et al. (2020) Ventilation cooling/heating performance of a PCM enhanced ventilated window—An experimental study. Energy and Buildings, 214, p. 109903. doi.org/10.1016/j.enbuild.2020.109903.
- Foteinaki, K., et al. (2018) Heating system energy flexibility of low-energy residential buildings. Energy and Buildings, 180, pp. 95–108. doi.org/10.1016/j.enbuild.2018.09.030.
- Jiang, C., et al. (2018) Exploring the Demand Side Flexibility of a New Residential Building. In Proceedings of the 2nd International Symposium on Computer Science and Intelligent Control; Association for Computing Machinery (ACM), Stockholm, Sweden, 21–23 September ; p. 48.
- Johra, H., et al. (2019) Influence of envelope, structural thermal mass and indoor content on the building heating energy flexibility. Energy and Buildings, 183, pp. 325–339. doi.org/10.1016/j.enbuild.2018.11.012.
- Liu, M., et al. (2019) Energy flexibility of office buildings—Potential of different building types. E3S Web of Conferences, 111, p. 111.
- Wittchen, K.B., et al. (2020) Analyses of Thermal Storage Capacity and Smart Grid Flexibility in Danish Single-Family Houses. In Proceedings of the International Conference Organised by IBPSA-Nordic; Oslo, Norway, 13–14 October 2020, OsloMet: Oslo, Norway.
- Kolokotroni, M & Heiselberg, P K (2015) Ventilative Cooling—State-of-the-Art Review. Department of Civil Engineering, Aalborg University: Aalborg, Denmark.
- Foldbjerg, P., et al. (2014) Ventilative Cooling of Residential Buildings—Strategies, Measurement Results and Lessons Learned from Three Active Houses in Austria, Germany and Denmark. International Journal of Ventilation, 13, pp. 179–192.
- Lendager Arkitekter ApS (2021) The Resource Rows. Available at: https://lendager.com/
- Jessen, R.Z., et al. (2006) New Building Principles in Consequence of Legislative Demands for Reduced Energy Consumption in Danish Housing. In Proceedings of the PLEA2006—The 23rd Conference on Passive and Low Energy Architecture, Geneva, Switzerland, 6–8 September
- Dyrbøl, S., et al. (2005) Savings potential in existing Danish building stock and new constructions. In Proceedings of the ECEEE 2005 Summer Study—What Works & Who Delivers? Mandelieu la Napoule, France, 30 May–4 June; pp. 319–324.
- Wittchen, K.B & Kragh, J (2014) Energy Savings in the Danish Building Stock until 2050. In Proceedings of the NSB 2014: 10th Nordic Symposium on Building Physics, Lund, Sweden, 15–19 June.
- Jradi, M., et al. (2017) Dynamic Model-Driven Energy Retrofit of Bøgevangen and Runevej Daycare Centers in Aarhus. Energy Procedia, 132, pp. 975–981. doi.org/10.1016/j.egypro.2017.09.689.
- Jradi, M., et al. (2017) Deep energy renovation of the Mærsk office building in Denmark using a holistic design approach. Energy and Buildings, 151, pp. 306–319. doi.org/10.1016/j.enbuild.2017.06.047.
- Jradi, M., et al. (2018) Deep Energy Retrofit vs. Improving Building Intelligence: Danish Case Study. In Proceedings of the 2018 Building Performance Modeling Conference and SimBuild co-organized by ASHRAE and IBPSA-USA, Chicago, IL, USA, 26–28 September; pp. 470–477.
- Morelli, M., et al. (2012) Energy retrofitting of a typical old Danish multi-family building to a “nearly-zero” energy building based on experiences from a test apartment. Energy Building, 54, pp. 395–406. doi.org/10.1016/j.enbuild.2012.07.046.
- Odgaard, T., et al. (2018) Interior insulation—Characterisation of the historic, solid masonry building segment and analysis of the heat saving potential by 1d, 2d, and 3d simulation. Energy Building, 162, pp. 1–11. doi.org/10.1016/j.enbuild.2017.12.008.
- Odgaard, T., et al. (2015) Building Renovation with Interior Insulation on Solid Masonry Walls in Denmark—A study of the Building Segment and Possible Solutions. Energy Procedia, 78, pp. 830–835. doi.org/10.1016/j.egypro.2015.11.003.
- Foldbjerg, P., et al. (2014) Indoor Climate in a Danish Kindergarten built according to Active House Principles: Measured Thermal Comfort and use of Electrical Light. In Proceedings of the AIVC conference, Poznan, Poland, 24–25 September; pp. 188–197.
- Larsen, T.S., et al. (2012) The Comfort Houses: Measurements and Analysis of the Indoor Environment and Energy Consumption in 8 Passive Houses 2008–2011; Department of Civil Engineering, Aalborg University: Aalborg, Denmark.
- Sørensen, M.J., et al. (2017) Integrated Building Energy Design of a Danish Office Building Based on Monte Carlo Simulation Method. Energy Procedia, 132, pp. 93–98.
- Vanhoutteghem, L., et al. (2015) Impact of façade window design on energy, daylighting and thermal comfort in nearly zero-energy houses. Energy Building, 102, pp. 149–156. doi.org/10.1016/j.enbuild.2015.05.018.
- Vanhoutteghem, L &Svendsen, S (2014) Modern insulation requirements change the rules of architectural design in low-energy homes. Renewable Energy, 72, pp.301–310. doi.org/10.1016/j.renene.2014.07.005.
- Montana, F., et al. (2020) Multi-Objective Optimization of Building Life Cycle Performance. A Housing Renovation Case Study in Northern Europe. Sustainability, 12, p. 7807. doi.org/10.3390/su12187807.
- Jørgensen, O B &Hendriksen, O J (2000) Glazed Balconies and Sun Spaces–Energy Savers or Energy Wasters? In Proceedings of the Third ISES Europe Solar Congress (Eurosun2000), Copenhagen, Denmark, 19–22 June.
- Van Uffelen C & Bayandin, A (2009) Ecological Architecture, 1st ed.; Heinel, M., Saupe, N., Eds.; Braun Publishing AG: Salenstein, Switzerland.
- VELUX Group. Solhuset, Denmark—A Kindergarten with a Sustainable Difference. Available at: https://velcdn.azureedge.net/-/media/marketing/master/professional/cases/solhuset_denmark/120541-01_r579-005.pdf .
- Psomas, T., et al. (2016) Overheating risk barriers to energy renovations of single family houses: Multicriteria analysis and assessment. Energy Building, 117, pp. 138–148. doi.org/10.1016/j.enbuild.2016.02.031.
- Skarning, G. C. J., et al. (2017) The effect of dynamic solar shading on energy, daylighting and thermal comfort in a nearly zero-energy loft room in Rome and Copenhagen. Energy Building,135, pp. 302–311. doi.org/10.1016/j.enbuild.2016.11.053.
- Karlsen, L., et al. (2016) Solar shading control strategy for office buildings in cold climate. Energy Building, 118, pp. 316–328. doi.org/10.1016/j.enbuild.2016.03.014.
- Nielsen, M.V., et al. (2011) Quantifying the potential of automated dynamic solar shading in office buildings through integrated simulations of energy and daylight. Solar Energy, 85, pp. 757–768. doi.org/10.1016/j.solener.2011.01.010.
- Noergaard, T (2018) A Human Sense of Architecture. In Sustainability in Scandinavia—Architecture Design and Planning, Edition Axel Menges: Fellbach, Germany.
- Oropeza-Perez, I & Østergaard, P A (2014) Potential of natural ventilation in temperate countries—A case study of Denmark. Applied Energy, 114, pp. 520–530. doi.org/10.1016/j.apenergy.2013.10.008.
- Psomas, T., et al. (2016) Control Strategies for Ventilative Cooling of Overheated Houses. In Proceedings of the CLIMA 2016—12th REHVA World Congress, Aalborg, Denmark, 22–25 May 5.
- Gao, J., et al. (2014) Ventilation system type, classroom environmental quality and pupils’ perceptions and symptoms. Building and Environment, 75, pp. 46–57. doi.org/10.1016/j.buildenv.2014.01.015.
- Toftum, J., et al. (2015) Association between classroom ventilation mode and learning outcome in Danish schools. Building and Environment, 92, pp. 494–503. doi.org/10.1016/j.buildenv.2015.05.017.
- Tranholm, G.T., et al. (2012) Reducing energy consumption in an existing shopping centre using natural ventilation. In Proceedings of the 33rd AIVC- 2nd TightVent Conference, Copenhagen, Denmark, 10–11 October.
- Bogatu, D.-I., et al. (2019) A Preliminary Analysis on the Night Cooling Potential of Photovoltaic/thermal (PV/T) Panels for European Cities. E3S Web of Conferences, 111, p. 111. doi.org/10.1051/e3sconf/201911101055.
- Bourdakis, E., et al. (2016) Simulation Study of Discharging PCM Ceiling Panels through Night-time Radiative Cooling. In Proceedings of the ASHRAE Annual Conference [ST-16-C011], St. Louis, MO, USA, 26 June.
- Pean, T.Q., et al. (2016) Influence of the environmental parameters on nocturnal radiative cooling capacity of solar collectors. In Proceedings of the CLIMA2016—12th REHVA World Congress, Aalborg, Danmark, 22–25 Ma.
- Pean, T. Q., et al. (2015) Nighttime radiative cooling potential of unglazed and PV/T solar collectors: Parametric and experimental analyses. In Proceedings of the 8th Mediterranean Congress of Heating, Ventilation and Air-Conditioning (CLIMAMED), Nice, France, 9–11 September.
- Hviid, C.A., et al. (2020) Cooling of schools—results from a demonstration project using adiabatic evaporative cooling with harvested rainwater. E3S Web of Conferences, 172, p. 02003. doi.org/10.1051/e3sconf/202017202003.
- Pomianowski, M., et al. (2015) Technical Potential of Evaporative Cooling in Danish and European Condition. Energy Procedia, 78, pp. 2421–2426. doi.org/10.1016/j.egypro.2015.11.211.
- European Commission. Renovation Wave. Available at: https://ec.europa.eu/energy/topics/energy-efficiency/energy-efficient-buildings/renovation-wave_en.
- Artmann, N., et al. (2007) Climatic potential for passive cooling of buildings by night-time ventilation in Europe. Applied Energy, 84, pp. 187–201. doi.org/10.1016/j.apenergy.2006.05.004.
- Fabi, V., et al. (2012) Main physical environmental variables driving occupant behaviour with regard to natural ventilation. In Proceedings of the 5th International Building Physics Conference, Kyoto, Japan, pp. 28–31 May.
- Chen, Y., et al. (2017) Investigating natural ventilation potentials across the globe: Regional and climatic variations. Building and Environment, 122, pp. 386–396. doi.org/10.1016/j.buildenv.2017.06.026.