By Nidhi DhullReviewed by Susha Cheriyedath, M.Sc.Apr 10 2024
Concrete is the second most extensively used material after water, and it finds applications in buildings, roads, pavements, pipelines, nuclear power plants, dams, etc. Several advances have been made towards producing strong, lightweight, durable, and recyclable concrete. However, cracking, degradation, deformation, and scaling issues persist due to long-term environmental and mechanical causes, which decrease the life of concrete structures.1
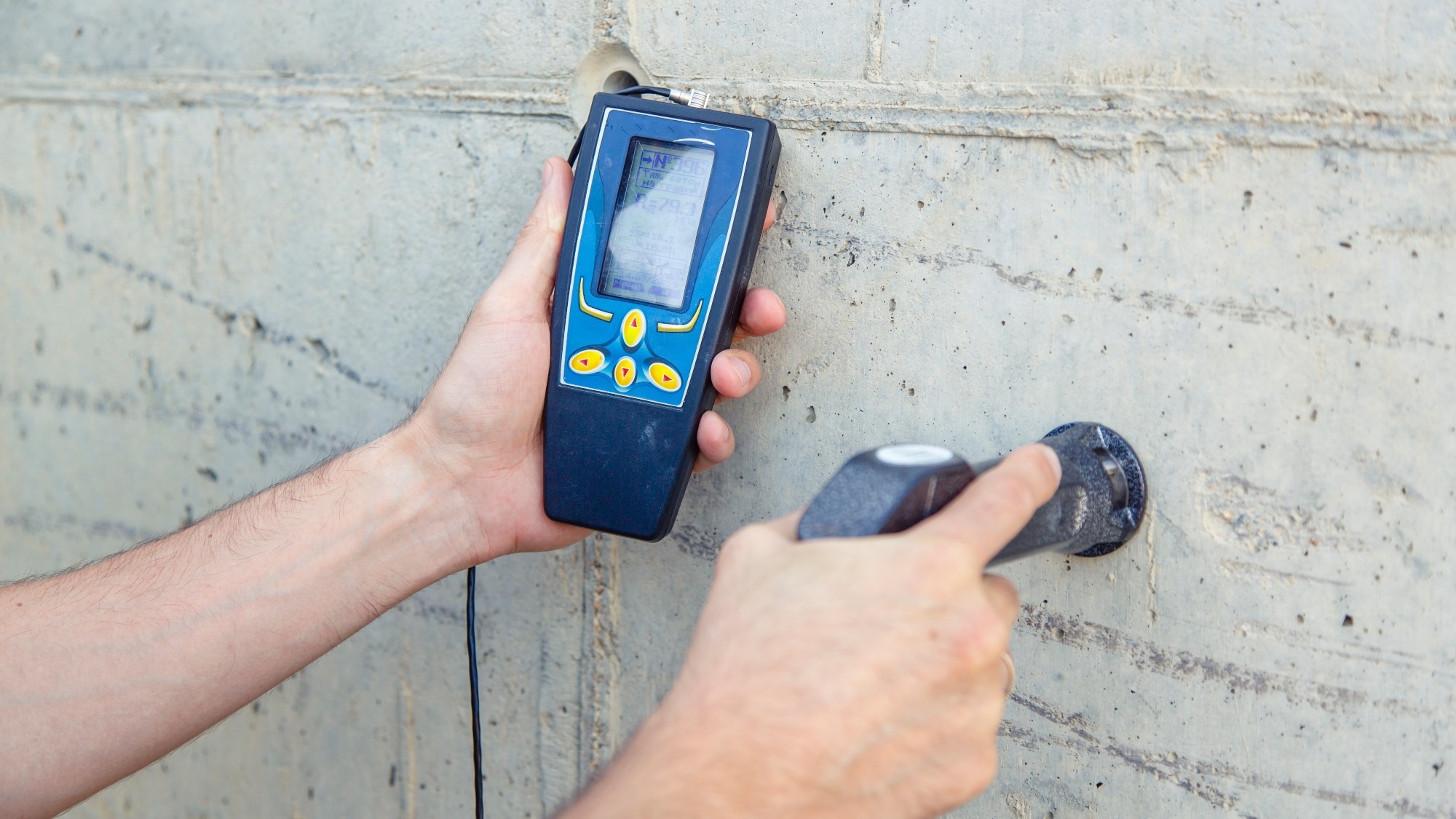
Image Credit: AleksNT/Shutterstock.com
Recent developments in concrete sensor technology can help overcome these challenges.
Concrete sensors are embedded within the reinforcement array to monitor parameters like temperature and humidity in real-time.2 Additionally, self-sensing concrete is being developed to sense and monitor concrete structures.1 This article explores the advantages, applicability, and challenges of concrete sensor technology.
Advantages of Concrete Sensor Technology
Self-sensing concrete can enhance safety, serviceability, durability, and reliability by constantly sensing and monitoring the concrete structures.
Functional fillers like carbon black, carbon fibers, nanomaterials, glass fiber, and graphite powder incorporated into normal concrete improve its mechanical characteristics and impart the ability to sense stress, strain, or damage. These functional fillers exhibit a piezoresistive effect and are dispersed in the matrix phase of concrete production to produce a conducting network. When an external force is applied, the electrical resistance of the concrete changes, thereby facilitating self-sensing capabilities within the concrete.1
Traditional sensors used in structural health monitoring, like accelerometers, gyroscopes, strain gauges, velocimeters, and high-resolution cameras, are typically applied to small sections of structures and come with complex installation procedures and significant costs.3 In contrast, sensors embedded in concrete present several benefits, including low cost, straightforward installation and maintenance, extended service life, dependable structural properties, and high sensitivity.1
Multifunctional self-sensing concrete can be integrated into entire structures, enabling these structures to autonomously monitor their behavior and integrity.3 The data collected by the embedded concrete sensors can be accessed via an external system, using both wired and wireless methods of connection.2
Applications of Concrete Sensors
Self-sensing concrete is utilized in structural health monitoring to detect damage resulting from corrosion, strain, seismic activities, and other factors.
Concrete sensors are being used to develop smart bricks capable of tracking minor strain variations in masonry buildings. Additionally, if the concrete is resistant to variations in temperature and humidity, the smart bricks can serve dual functions as fire alarms and moisture detectors.1 In the event of catastrophic disasters like earthquakes, concrete sensors can also facilitate a rapid evaluation of structural health in the affected area, thereby supporting prompt evacuation and rescue efforts.3
Additionally, concrete sensors find application in tracking the operational health of diverse infrastructure systems. This includes leak detection in pipelines and tracking corrosion inside concrete components. Another key application of concrete sensor technology lies in traffic monitoring, where it is capable of detecting traffic flows and sensing the weight of moving vehicles through the use of piezoelectric composites.1 Ultimately, the integration of concrete sensors promotes cost-effective and efficient practices in the design, construction, and management of transportation infrastructures.5
Challenges in Concrete Sensor Technology
The sensing abilities of concrete are significantly influenced by several key factors: the quantity and type of functional filler used, how this filler is distributed, its components, and its physical attributes (like size, shape, surface texture, and the degree of its clumping together).1 The sensitivity of concrete sensors hinges on the piezoresistance of these fillers, the electrical properties of the matrix, how well the filler integrates with the matrix, and how compatible the concrete is with the electrodes.3 Modifying concrete to enhance its sensing capabilities requires a meticulous evaluation of all these elements to ensure optimal performance.
Achieving a uniform distribution of functional fillers is essential for maintaining the concrete's uniformity and for the effective formation of an electrical network within the matrix.1 However, the performance of concrete sensors can be impacted by the presence of coarse aggregates and steel rebars in reinforced concrete. Among various dispersion techniques, sonication stands out for its efficiency, particularly with nano-sized fillers. Despite its effectiveness, scaling up this method to accommodate large-scale infrastructure projects remains a challenge.3
The selection of concrete sensors is guided by their respective advantages and limitations, with the decision hinging on the specific application and the expected return on investment for the project. For example, while commercially available concrete thermocouples are cost-effective, they suffer from low accuracy in measurements. Additionally, their wiring requires extra protection in challenging construction environments. Similarly, the use of wired-temperature and maturity loggers introduces labor-intensive challenges.2
Moreover, the dynamic sensing capabilities of concrete sensors are compromised by instrumentation noise, which arises from the complexity of integrating multiple high-impedance electrical circuits. To overcome these obstacles and harness concrete sensors for advanced engineering applications, there is a crucial need for improvements in electronic hardware components. Furthermore, the development of smart, multifunctional materials with enhanced strain sensitivity is essential for optimizing the performance of concrete sensors in advanced engineering applications.3
Latest Developments
The integration of wireless technology with concrete sensor technology has resolved the above-mentioned shortcomings. Fully embedded wireless concrete sensors use Bluetooth, Wi-Fi, low power long range (LoRa), and long-term evolution (LTE) communication routes to send recorded data to external computers or mobile applications.2,5
Advancements in computing and modeling have the potential to significantly expedite the commercialization process of concrete sensor technology, offering considerable savings in both time and financial investments.
Through the application of computational methods, the selection of functional fillers, optimization of dispersion methods, integration of additional concrete components, determination of the most effective sensing methods, and thorough performance analysis of sensors can be efficiently conducted in the pre-production phase.3 This strategic approach not only streamlines the development process but also enhances the decision-making framework, ensuring a more effective and economically viable deployment of concrete sensor technology.
Another crucial development in concrete sensor technology is the use of fiber optic sensors (FOS) to produce smart concrete. FOS address many of the limitations associated with electrical sensors, such as their restricted sensitivity, instability, and susceptibility to corrosion.
The advantages of FOS, including their lightweight nature, durability, resistance to chemical damage, and capabilities for remote and distributed sensing, position them as compelling alternatives to piezoresistive fillers in concrete. Various types of FOS, including grating, fluorescence-based, surface plasmon resonance, and distributed FOS, offer the potential for continuous monitoring of critical parameters such as temperature, moisture, chemical infiltration, corrosion, and pH levels in concrete structures, enhancing the longevity and safety of these constructions.4
Conclusion and Future Prospects
The next wave of innovations in concrete sensor technology is likely to be driven by advancements in cloud computing, machine learning algorithms, and artificial intelligence (AI)-assisted data analysis. These technologies promise to broaden the range of applications for smart concrete, including the ability to visually display temperature and strength profiles.
For instance, embedded concrete sensors integrated with rapid data acquisition and communication systems can be employed to determine the optimal traffic opening time.5 This integration of cutting-edge technologies with concrete sensor systems is poised to enhance the efficiency, safety, and functionality of infrastructure projects, transforming the way we monitor and manage the structural health of built environments.
Concrete sensors with real-time and in situ strength monitoring capabilities are expected to significantly reduce infrastructure project costs with accelerated construction schedules. The two most used laboratory tests for concrete strength, cylinder testing, and maturity curves, could also be replaced by on-site concrete sensors. These sensors offer greater precision in measuring concrete's strength.
This advancement not only promises to streamline construction processes but also contributes to environmental sustainability by reducing the carbon footprint of concrete structures. This is achieved by eliminating the need for additional cement that is often added to ensure strength, addressing discrepancies between laboratory-based and onsite strength analyses.6
In conclusion, intelligent, autonomous, sustainable, and resilient infrastructures with smart sensing capabilities could become a reality in the future, thanks to concrete sensing technology.6
References and Further Reading
1. Bekzhanova, Z., Memon, S. A., & Kim, J. R. (2021). Self-Sensing Cementitious Composites: Review and Perspective. Nanomaterials, 11(9), 2355. https://doi.org/10.3390/nano11092355
2. Concrete Sensors: Working and Types (2022). The Constructor. https://theconstructor.org/concrete/concrete-sensors-working-and-types/561638/
3. D’Alessandro, A., Birgin, H. B., & Ubertini, F. (2022). Carbon Microfiber-Doped Smart Concrete Sensors for Strain Monitoring in Reinforced Concrete Structures: An Experimental Study at Various Scales. Sensors, 22(16), 6083–6083. https://doi.org/10.3390/s22166083
4. Qiao, H., Lin, Z., Sun, X., Li, W., Zhao, Y., & Guo, C. (2023). Fiber Optic-Based Durability Monitoring in Smart Concrete: A State-of-Art Review. Sensors, 23(18), 7810–7810. https://doi.org/10.3390/s23187810
5. Kong, Z., & Lu, N. (2023). Determining Optimal Traffic Opening Time Through Concrete Strength Monitoring: Wireless Sensing. Purdue E-Pubs (Purdue University). https://doi.org/10.5703/1288284317613
6. Cardno, C. A. (2021). Technology: In situ concrete maturity sensors save construction time and money. American Society of Civil Engineers. https://www.asce.org/publications-and-news/civil-engineering-source/civil-engineering-magazine/issues/magazine-issue/article/2021/04/in-situ-concrete-maturity-sensors-save-construction-time-money
Disclaimer: The views expressed here are those of the author expressed in their private capacity and do not necessarily represent the views of AZoM.com Limited T/A AZoNetwork the owner and operator of this website. This disclaimer forms part of the Terms and conditions of use of this website.